BLAST-TNG: Tracing Magnetic Fields Across Our Galaxy
Our universe is permeated by magnetic fields. Just as the Earth’s magnetic field can alter the trajectory of charged particles that would otherwise hit our planet, the magnetic field of our galaxy can direction the motion of charged particles along magnetic field lines.
One area where magnetic fields likely play an important physical role is in the process of star formation. Stars form in clouds of mostly molecular gas. Because the clouds are penetrated by cosmic rays, some small fraction of the gas particles will be ionized. The presence of this ionized gas means that the gas in these star forming regions will be coupled to the magnetic field, and if the magnetic field is sufficiently strong it can slow down or stop the gravitational collapse of gas into stars.
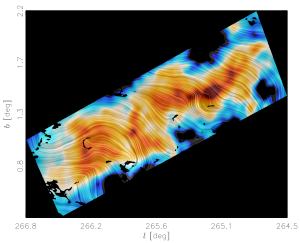
Figure 1. A magnetic field map of the Vela C star-forming region made with BLAST-TNG’s predecessor BLASTPol (Fissel et al., 2016) observing at 500 microns. The background image shows the light emitted by the dust grains, which shows the structure of the cloud. The “streaks” overlaid on top of the image show the orientation of the magnetic field inferred from BLASTPol data.
BLAST-TNG is designed to make incredibly detailed maps of magnetic fields. It has been known since the 1940s that interstellar dust grains tend to align on average perpendicular to their local magnetic field. The polarization direction of the light they emit can be used to infer the net alignment of the grains. By observing the polarized light emitted by these small (<0.001mm) dust grains, BLAST can therefore map magnetic fields in distant star forming regions. Because BLAST operates above 99.5% the atmosphere (which absorbs most of the radiation in the far-infrared and submm), it can make larger and more sensitive maps than telescopes of the same size on the ground (for example see Figure 1).
While these maps give an incredibly detailed portrait of the magnetic field, they don’t directly tell us the magnetic field strength. To understand whether the magnetic field is sufficiently strong to affect the motions of the gas and possibly slow down star formation, we need to compare our observations of the magnetic field to “synthetic maps” of magnetic fields derived from computer simulations of star forming regions such as the ones shown in Figure 2. In strong field simulations the magnetic field shows considerably more order than in weak magnetic field simulations. Also while in weak magnetic field simulations the cloud density structures align on average parallel to the field, in strong-field simulations the low density features tend be oriented parallel to the field, while the higher density features tend to be perpendicular to the field.
Strong magnetic field (|B0|=10.97 μG) | Weak magnetic field (|B0|=0.35μG) |
In addition because the efficiency with which dust grains are able to emit polarized light depends on their shape, composition and ability to line with the local magnetic field, studying polarized light from dust grains can be used to learn about dust grain properties (e.g. Draine and Fraisse 2009).
Our main science goals for our upcoming 2017 Antarctic Science Flight are to:
- Map magnetic fields in the nearest star forming regions (distance < 3000 light years), and characterize the magnetic field strength in each region through detailed comparisons with simulations (read more: Nearby Molecular Clouds);
- Map dozens of more distant massive star forming clouds to study how magnetic fields affect the formation and evolution of star forming regions (read more: Galactic Plane/Giant Molecular Cloud Science);
- Study magnetic fields and dust properties in the more diffuse regions of our galaxy (read more: Diffuse ISM Galactic Science).
- Investigate whether polarized radiation from dust grains in the diffuse regions of our galaxy can contaminate other astronomical observations, such as measurements of the cosmic microwave background (read more: Diffuse ISM CMB Foregrounds);
- Study magnetic fields in other nearby galaxies (read more: Other Galaxies).
BLAST-TNG polarization maps will be made publicly available shortly after publication. In addition we will be making available 25% of our science time to the astronomy community for shared risk observations. (See Information for Observers.)